UNIT - 9 Coordination Compounds
Learning Outcomes
The learners will be able to
-
know the postulates of Werner’s theory of Coordination Compounds
-
understand the definations of central atom/ions, ligand, coordination number, oxidation number, coordination sphere, hamolepitc and heteroleptics
-
learn and write the nomenclature of mononuclear coordination compounds
-
explain the different types of isomerism in coordination compounds
-
know the nature of bonding in coordination compounds in terms of valence bond and crystal field theories
-
Double salts: These are the molecular compounds which exist only in the solid state and lose their identity when dissolved in water, i.e., they dissociate into simple ions completely when dissolved in water. In such compounds the individual properties of the constituent particles are not lost. For example aqueous solution of potash alum will give the tests for
, and ions. Some well known double salts are
Potash alum
Carnallite
Mohr’s salt
-
Coordination or complex compounds: These are the molecular compounds which retain their identity in the solid as well as in the dissolved state and their properties are completely different from those of their consituent particles. In aqueous solution, these compounds do not furnish all simple ions but instead give complex ions. For example, potassium argentocyanide does not form
and simpler ions but instead give argentocyanide complex ion (which does not dissociate) -
Cationic complexes: A complex in which the complex ion carries a net positive charge is called cationic complex, e.g.,
-
Anionic complexes: A complex in which the complex ion carries a net negative charge is called anionic complex, e.g.,
-
Neutral complexes: A complex carrying no net charge is called a neutral complex or simply a complex e.g., [Ni
Coordination entity: Central atom / ion (metal) to which a fixed number of other atoms or groups are attached (ligand).
Coordination entity can be neutral or charged e.g.
- Ligand: An ion or molecule which is capable of donating a pair of electrons to the central atom is called a coordinating group or ligand. The atom in the ligand which donates the electron pair is called the donor atom or ligating group. The common donor atoms are
, and halogens. The ligands containing one, two or more donor atoms are known as unidentate, bidentate, or multidentate respectively.
Examples of bidentate (or didentate) ligands are:
Ethylenediamine (en) or (ethane-1,2-diamine),
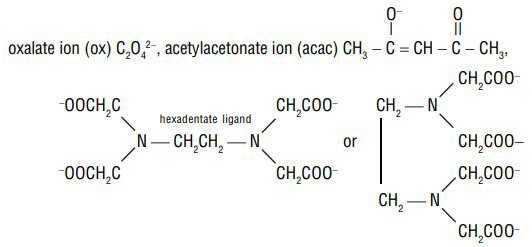
Ethylenediaminetetraacetate ion (edta
-
Denticity of a ligand: The number of donor atoms or ligating groups from a given ligand attached to the same central metal atom is called the denticity of the ligand.
-
Chelating ligands: When a multidentate ligand simultaneously coordinates to a metal ion through more than one sigma electron pair donor site or group, a ring like structure is obtained. It is called a chelate and the ligand is known as chelating ligand. The process of formation of rings is called chelation.
-
Coordination sphere
Counter Ions: The central atom/ion and the ligands attached to it are enclosed in square bracket and is collectively termed as coordination sphere and the ionizable groups written outside the coordination sphere is called counter ions etc. In
-
Coordination number: of the central atom/ion is the number of sigma bonds between the ligands and the central atom/ion.
-
pi bonds not considered for coordination number.
-
Coordination polyhedra: the spatial arrangement of the ligand atoms which are directly attached to the central atom / ion.
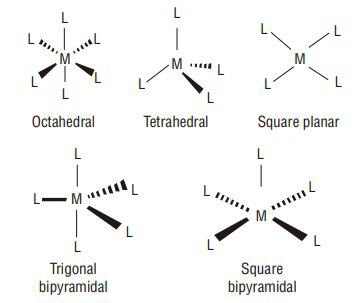
-
Oxidation number of central atom: The charge that central atom/ion would carry if all the ligands are removed along with the electron pairs that are shared with the central atom.
-
Chelate effect: The stability of complexes is greatly increased by chelation. This is known as the chelate effect. The more the number of rings present in a complex, the more the stablity it acquires. For example
is less stable as it has only two rings in the structure. One the other hand, [Fe(edta) is more stable as it contains five rings in the structure. -
Ambidentate ligands: Unidentate ligands containing more than one coordinating atoms e.g.,
can coordinate through as well as through is well as . -
Homoleptic and heteroleptic complexes: Complexes in which the central metal atom or ion is linked to only type of ligands are called homoleptic complexes. For example,
. The complexes in which the central metal atom or ion is linked to more than one kind of ligands are called heteroleptic complexes. For example, . -
Homonuclear and polynuclear complexes: Complexes in which only one metal atom is present are known as homonuclear complexes. On the other hand, the complexes in which more than one metal atom is present are known as polynuclear complexes.
Werner’s Theory of Coordination Compounds
Alfred Werner, a Swiss chemist proposed a theory about the nature of bonding in the coordination compounds (complexes) in the year 1893. The main postulates of this theory are:
- Metals possess two types of linkages (valencies).
a) Primary or pinicipal or ionizable links (valency) which are the same as oxidation state of the metal.
b) Secondary or non-ionizable links (valency) which are the same as co-ordination number of the central metal atom/ion. This number is fixed for a metal.
-
A metal atom satisfies both primary as well as secondary linkages (valencies). Primary linkages (valencies) are satisfied by negative ions whereas secondary linkages (valencies) are satisfied by ligands (neutral or negative ions/groups).
-
Ligands satisfying secondary linkages (valencies) are directed towards fixed directions (spatial arrangements) giving a definite geometry to the complex but primary valencies are nondirectional. Such spatial arrangements are called coordination polyhedra. Werner’s theory could not explain why
i) only certain elements possess the remarkable property of forming coordination compounds?
ii) the bonds in coordination compounds have directional characteristics?
iii) coordination compounds have characteristic magnetic and optical properties?
Examples of ligand
Name | Formula | Charge | Donor atom/ atoms | Name given in the complex |
---|---|---|---|---|
Negative ligands | ||||
(a) Unidentate | ||||
Cyanide ion | -1 | cyano or cyanido | ||
Halide ion | -1 | halido (flourido/ chlorido/bromido/ iodido) |
||
Hydride | -1 | hidrido | ||
Nitro | -1 | nitrito-N | ||
Nitrite ion | ONO- | -1 | 0 | nitrito-O |
Nitrate ion | -1 | nitrato | ||
Hydroxide ion | -1 | hydroxo | ||
Amide ion | -1 | amido | ||
Thiocyanate ion | -1 | thiocyanato | ||
Isothiocyanate ion | NCS |
-1 | isothiocyanato | |
Acetate ion | -1 | acetato | ||
Oxide ion | -2 | oxo | ||
Peroxide ion | -2 | peroxo | ||
Sulphide ion | -2 | sulphido | ||
Sulphite ion | -2 | sulphito | ||
Sulphate ion | -2 | sulphato | ||
thiosulphate ion | -2 | thiosulphato | ||
Carbonate ion | -2 | carbonato | ||
Imide ion | -2 | imido |
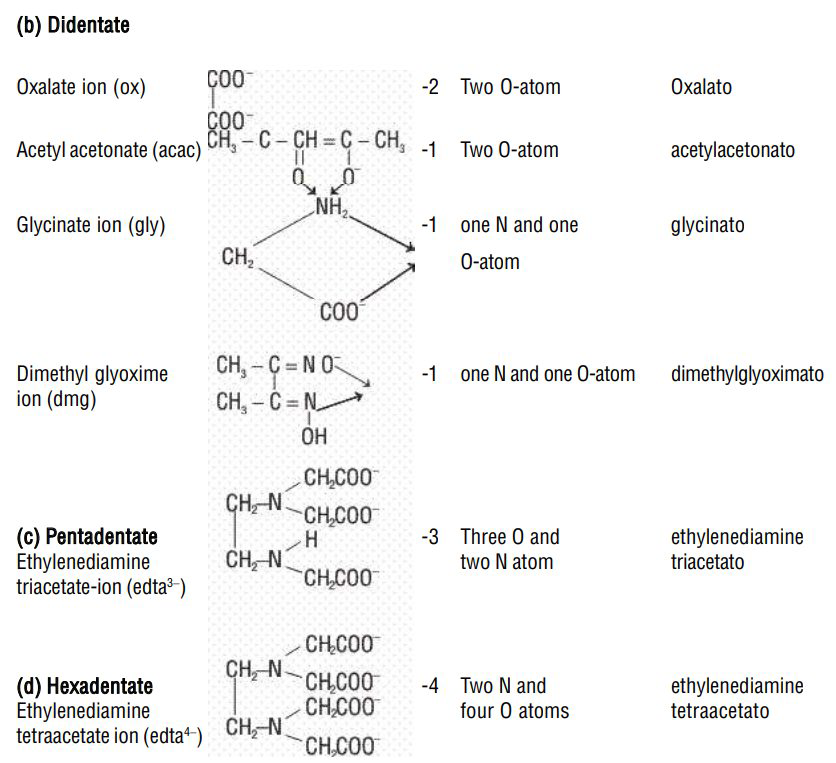
Neutral Ligands
a) Unidentate
Name | Formula | Charge | Donor atom/ atoms | Name given in the complex |
---|---|---|---|---|
Methylamine | zero | Methylamine | ||
Ammonia | zero | ammine | ||
Water | zero | 0 | aqua | |
Nitric oxide | NO | zero | nitrosyl | |
Carbon monoxide | zero | 0 | carbonyl | |
Thiocarbonyl | cS | zero | thiocarbonyl | |
Phosphine | zero | P | phosphine | |
Triphenyl phosphine | zero | P | triphenyl phosphine | |
Thiourea (tu) | ![]() |
zero | S | thiourea |
Pyridine (py) | ![]() |
zero | pyridine |
b) Bidentate
Name | Formula | Charge | Donor atom/ atoms | Name given in the complex |
---|---|---|---|---|
Ethylenediamine (en) | ![]() |
zero | Two |
ethylene diamine |
2.2-Dipyridv1(dipy) | ![]() |
zero | Two |
dipyridyl |
Ortho-ohenanthroline | ![]() |
zero | Two |
phenonanthroline |
c) Tridentate
Name | Formula | Charge | Donor atom/ atoms | Name given in the complex |
---|---|---|---|---|
Diethylene triamine (dien) | ![]() |
zero | Three N atoms | diethlene tetramine |
d) Tetradentate
Name | Formula | Charge | Donor atom/ atoms | Name given in the complex |
---|---|---|---|---|
triethylene tetramine (trien) | ![]() |
zero | Four N atoms | triethylene tetramine |
Positive Ligands
Name | Formula | Charge | Donor atom/ atoms | Name given in the complex |
---|---|---|---|---|
Hydrazinium ion | +1 | hydrazinium | ||
Nitrosonium ion | +1 | nitrosonium | ||
Nitronium ion | +1 | nitronium |
Rules for naming mononuclear coordination compounds
1. Order of naming ions
The positive ion (cation) whether simple or complex, is named first followed by the negative ion (anion). The name is started with a small letter and the complex part is written as one word.
2. Naming ligands
i) Negative ligands (organic or inorganic) end in
If the name of the anionic ligands ends in -ide, -ite or -ate, the last ’
ii) Neutral ligands have no special ending,
iii) Positive ligands (which are very few) end in -ium,
3. Numerical prefixes to indicate number of ligands
If there are several ligands of the same type, the prefixes like di, tri, tetra, penta and hexa are used to indicate the number of ligands of that type.
When the name of polydentate ligand includes a number e.g., ethylendediamine, then bis, tris, tetrakis are used as prefixes.
4. Order of naming of ligands
All ligands whether negative, neutral or positive are named first in the alphabetical order followed by the name of the metal atom/ion.
5. Naming of the complex ion and ending of the central atom
Ligands are named first followed by the metal atom.
a) If the complex ion is a cation or the coordination compund is non-ionic, the name of the central metal ion is written as such followed by its oxidation state indicated by Roman numeral (such as II, III, IV) in the parentheses at the end of the name of the metal without any space between the two.
b) If the complex ion is anion, the name of the central metal atom is made to end in-ate followed by the oxidation number in brackets without any space between them.
Rules for Writing Formula from the Name of the Mononuclear Complex
-
Formula of the cation (whether simple or complex) is written first followed by that of the anion.
-
The formula of the complex ion (coordination entity) (whether charged or not) is written in square brackets called coordination sphere.
-
Within the coordination sphere, the symbol of the metal atom is written first followed by the symbols/formulas of the ligands arranged alphabetically according to their names irrespective of the charge present on them.
While listing the ligands alphabetically, the following rules should be followed:
i) Polydentate ligands are also listed in the alphabetical order.
ii) The position of abbreviated ligands in the alphabetical order is determined from the first letter of the abbreviation.
iii) The position of ligands with special names (such as aqua for water) in the alphabetical order is determined from the first letter of the special name.
iv) Abbreviations used for the ligands and the formulas of the polyatomic ligands are enclosed in parentheses separately.
v) The metal atom as well as all the ligands are listed without any space between them.
-
If the formula of the complex ion is to be written without writing the counter ion, the charge on the complex ion is indicated outside the square bracket as a right superscript with the number before the sign. For example,
, etc. -
The number of cations or anions to be written in the formula is calculated on the basis that total positive charge must be equal to the total negative charge, as the complex as a whole is electrically neutral.
Example : Tetraammineaquachloridocobalt(III) chloride has complex ion
To balance the charge, the formula will be
ISOMERISM IN COORDINATION COMPOUNDS
Two or more chemical compounds which have identical chemical formula but different structures are known as isomers and the phenomenon is known as isomerism. The isomers have different arrangements of ligands around the central metal atom. It can be further classified into two types:
1) Structural Isomerism
a) Ionisation isomerism: Ionisation isomerism occurs when compounds with same molecular formula give different ions in the solution, for example,
b) Hydrate isomerism: The isomerism occurs when some ligand
c) Linkage isomerism: This isomerism takes place when a monodentate ligand has two possible donor atoms and attaches in two different ways to the central metal atom. Such ligands are known as also ambidentate ligands. For example, nitro
Linkage isomerism is found in
d) Coordination isomerism: This isomerism is shown by compounds in which both the cation as well as anion are complexes and ligands may interchange their positions between the two complex ions. Fo example,
2) Stereo-isomerism:
In stereo-isomerism, the isomers differ only in the spatial arrangement of atoms or groups about the central metal atom. It is also known as space isomerism. It can be further classified into two types:
a) Geometrical isomerism: This isomerism is due to the difference in geometrical arrangement of the ligands around the central atom. When similar ligands are on adjacent positions, it is known as cis form and when these are in the opposite positions, it is knowns as trans-form. Thus, this is also known as cis-trans isomerism.
- Geometrical isomerism in complexes with coordination number 4 : Tetrahedral complexes do not show this isomerism as the relative positions of the atoms, with respect to one another will be the same. Square planar complexes show geometrical isomerism. Compounds of the type
and show cis-trans isomersim. For example, [ , and show geometrical isomerism, In trans isomer, identical groups or ligands are situated across the central metal ion while in the cis isomer, the identical groups are situated on the same side of the central atom fixing the position of one of the ligands.
Square planar complexes (coordination number )
1. type
2. type
3. MABCD type
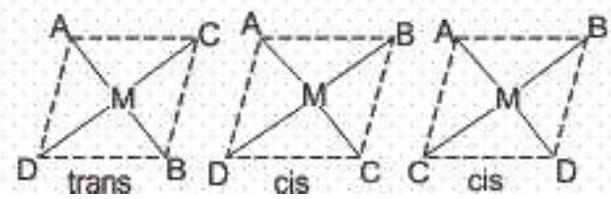
- Geometrical isomerism in complexes with coordination number 6:
Octahedral complexes of the type
Octahedral Complexes (Coordination number )
1. or type
2. or type
3. type
Facial and meridonial isomerism : Another type of geometrical isomerism occurs in octahedral coordination compounds of the type
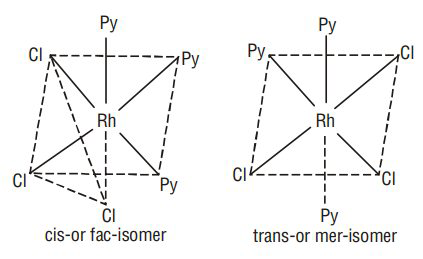
b) Optical Isomerism: The molecules which do not have a plane of symmetry (i.e., chiral molecules), exhibit optical isomerism. The optically active isomers known as enantiomers or enantiomorphs, are non superimposable mirror images of each other and rotate the plane of polarised light equally but in opposite directions. Those which rotate the plane towards the right are called dextro rotatory (d) or (+) while those which rotate towards the left are called laevorotatory (I) or (-). Complexes which have coordination numbers 4 and 6 show this type of isomerism.
Complexes in which at least one chelating ligands is present exist as a pair of enantiomers and have been isolated. Complexes of the type
Octahedral complexes of the type
1.
2.
3.
1.
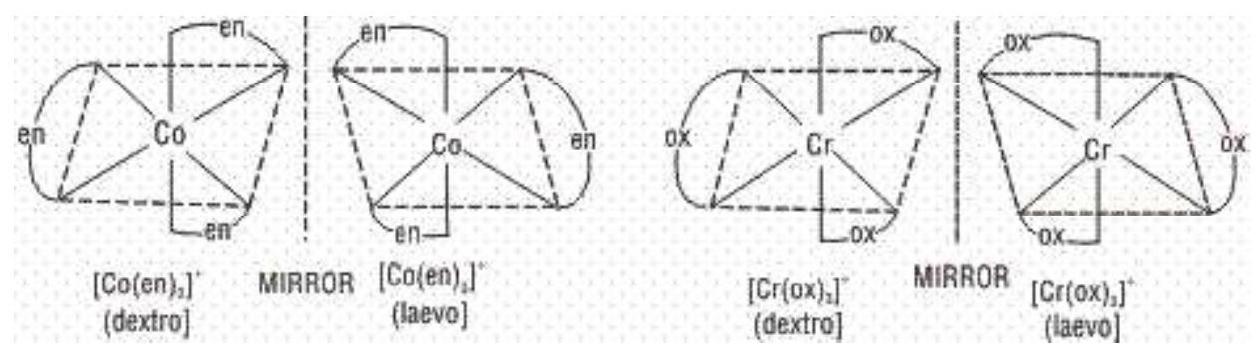
2.
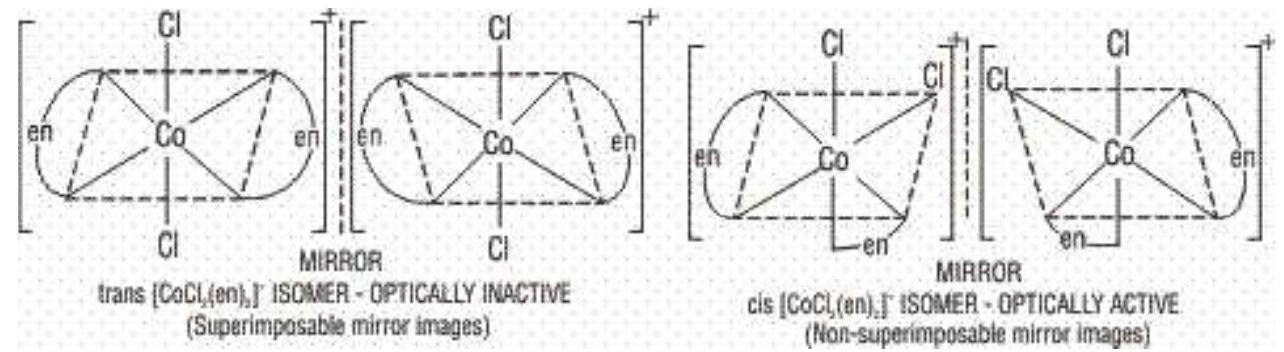
3.
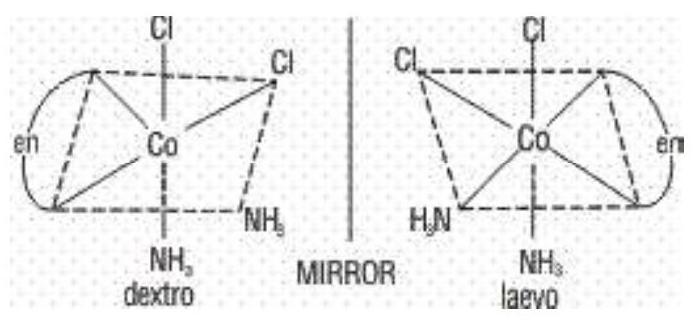
Solved Examples
1. Which one of the following has an optical isomer?
(en
Strategy: Optical isomerism is common in octahedral complexes involving 2 or 3 symmetrical bidentate groups.
Optical activity has been observed for chelated tetrahedral and square planar complexes but only rarely.
Show Answer
Solution:
Complex
Answer: (2)
2. Which among the following will be named as dibromidobis-(ethylenediamine) chromium (III) bromide?
Strategy: determine the number of
Show Answer
Solution: there are two
The charge on the complex is +1
The charge on the complex is balanced by the counter ion (Br)
Thus the complex is
Answer: (2)
PRACTICE QUESTIONS
Question 1- The correct name of the compound
(a) cuprammonium nitrate
(b) Tetrammonium copper (II) dinitrate
(c) tetraamminecopper (II) nitrate
(d) tetrammine copper (II) dinitrite
Show Answer
Answer:- cQuestion 2- The IUPAC name of the coordination compound
(a) potassium hexacyanoferrate (III)
(b) potassium hexacyanoferrate (II)
(c) tripotassium hexacyanoiron (II)
(d) potassium hexacyanoiron (II)
Show Answer
Answer:- aQuestion 3- The IUPAC name for the complex
(a) pentaammine nitrito-N-cobalt (II) chloride
(b) pentaammine nitrito-N-cobalt (III) chloride
(c) nitrito-N-pentaammine cobalt (III) chloride
(d) nitrito-N-pentaammine cobalt (II) chloride
Show Answer
Answer:- bQuestion 4- The IUPAC name of
(a) dichlorido (triphenyl phosphine) nickel(II)
(b) dichloridobis(triphenylphosphine) nickel(II)
(c) dichlorotriphenyl phosphine nickel (II)
(d) triphenylphosphine nickel (II) chloride.
Show Answer
Answer:- bQuestion 5- The IUPAC name of the following compound
(a) pentaamminecarbonatochromium(III) chloride
(b) pentaamminocarbonatochromium
(c) pentaamminocarbonatochromium(II) chloride
(d) pentaamminecarbonatochromium(IV) chloride
Show Answer
Answer:- aQuestion 6- Coordination number and oxidation number of
(a) 4 and +2
(b) 6 and +3
(c) 3 and +3
(d) 3 and 0
Show Answer
Answer:- bQuestion 7- Which of the following is a mixed salt?
(a)
(b)
(c)
(d)
Show Answer
Answer:- aQuestion 8- The ligand
(a) tridentate
(b) pentadentate
(c) tetradentate
(d) bidentate
Show Answer
Answer:- cQuestion 9- Which of the following is a negatively charged bidentate ligand?
(a) Dimethylglyximato
(b) Cyano
(c) Ethylenediamine
(d) Acetato
Show Answer
Answer:- aQuestion 10- One mole of the complex compound
(a)
(b)
(c)
(d)
Show Answer
Answer:- dQuestion 11- In the coordination compound
(a) 0
(b) +1
(c) +2
(d) -1
Show Answer
Answer:- aQuestion 12- The correct name for the complex
(a) hexaamminechromium (III) trioxalatocobalt (III)
(b) hexaamminechromiate (III) trioxalatocobalate (III)
(c) hexaamminechromium (III) trioxalatocobaltate (III)
(d) hexaamminechromate (III) trioxalatocobalt (III)
Show Answer
Answer:- cQuestion 13- Oxidation state of iron in
(a) +1
(b) +2
(c) +3
(d) +4
Show Answer
Answer:- bQuestion 14- Which of the following cannot show linkage isomerism?
(a)
(b)
(c)
(d)
Show Answer
Answer:- dQuestion 15- The oxidation states of
(a)
(b)
(c)
(d)
Show Answer
Answer:- dValence bond theory
According to valence bond theory, the metal atom or ion under the influence of ligands can use its (
1. Inner orbital octahedral complex- When the complex formed involved inner (
2. Outer orbital octahedral complex- When the complex formed uses outer d orbitals for hybridisation,
Coordination | Types of number hybridisation | Distribution of hybrid orbitals in space |
---|---|---|
4 | Tetrahedral | |
4 | Square planar | |
5 | Trigonal bipyramidal | |
6 | Octahedral | |
6 | Octahedral |
FORMATION OF OCTAHEDRAL COMPLEXES
1.
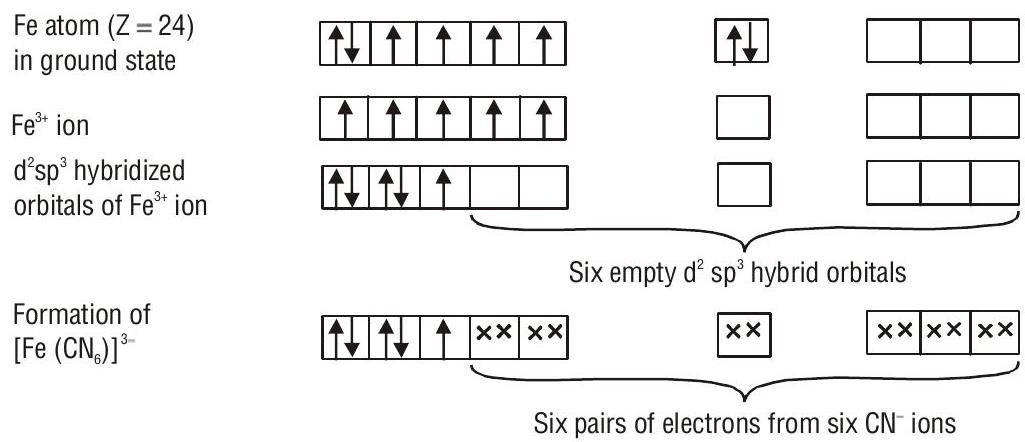
2.
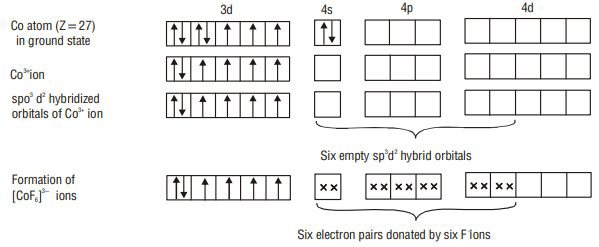
FORMATION OF SQUARE PLANAR COMPLEXES
2.
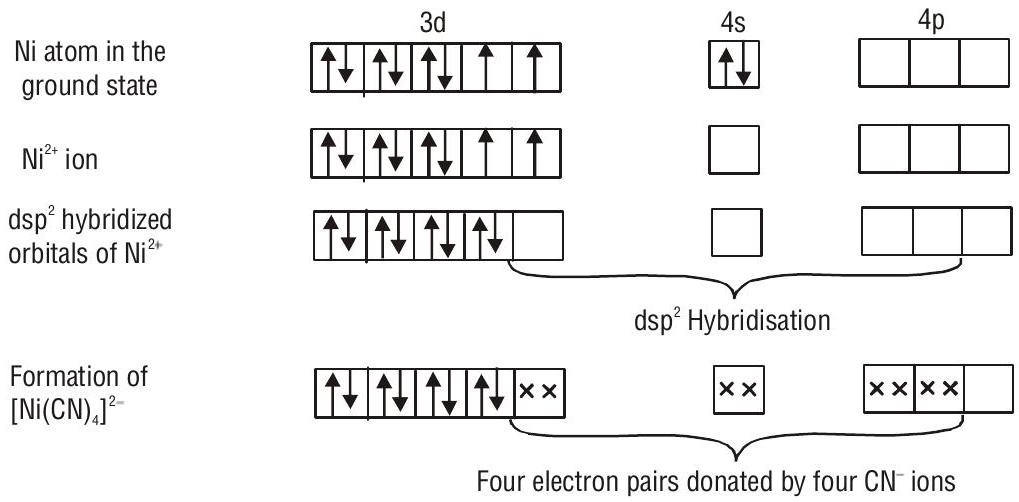
FORMATION OF TETRAHEDRAL COMPLEXES
1.
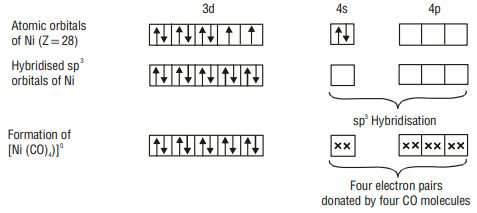
2.
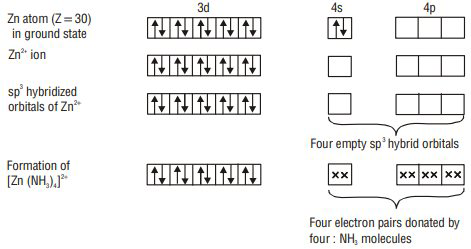
Limitations of Valence Bond Theory
The limitations of the valence bond theory are given as under:
i. It does not explain the relative energies of different structures.
ii. It offers no explanation of the colour observed for complex ion.
iii. It cannot predict whether a 4-coordinate complex will be tetrahedral or square planar.
iv. It does not take into account the splitting of d-energy levels.
v. It does not explain the spectra of complexes.
CRYSTAL FIELD THEORY
The splitting of five degenerate d-orbitals of metal ion under the influence of approaching ligands, into two sets of orbitals having different energies is called crystal field splitting. The magnitude of difference in energy between the two sets of orbitals
The magnitude of
Splitting of orbitals takes place in different manners in the case of tetrahedral and square planar complexes. In tetrahedral complexes, the splitting takes place in just the reverse manner. Here
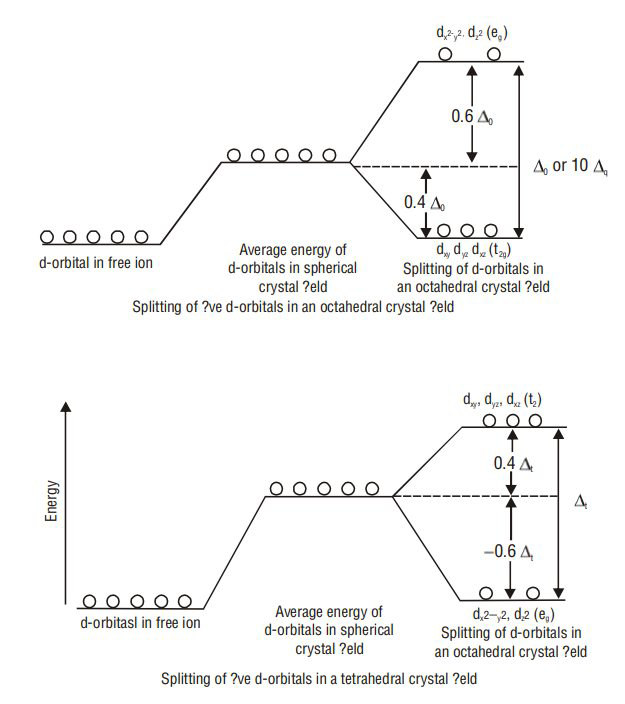
For configuration:
1. If
2. If
Applications of CFT
1. Magnetic behaviour
Paramagnetic- Unpaired electrons are present.
Diamagnetic- All electrons are paired.
2. Colours:
Now consider the complexes of cobalt with different ligands such as
The wavelengths absorbed will be in the opposite order as
Complex | |||
---|---|---|---|
Colour absorbed | Orange | Blue | Violet |
Colour transmitted | Blue | Yellow orange | Yellow |
Also the colour of the complex changes with the concentration of the ligand. To illustrate this, take
Reaction and colour changes | |
---|---|
ORGANOMETALLIC COMPOUNDS
These are the compounds in which a metal atom or a metalloid (
Types of Organometallic Compounds
Based on the nature of metal-carbon bond, they are classified into two types:
1.
2.
3.
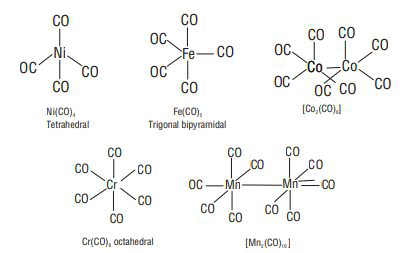
Bonding in metal carbonyls- M-C bonds in metal carbonyls have both
Solved Examples
Question 1- Which of the following facts about the complex
-
The complex involves
hybridization and is octahedral in shape. -
The complex is paramagnetic.
-
The complex is an outer orbital complex.
-
The complex gives white precipitate with silver nitrate solution.
Show Answer
Answer: (3)
Hint:
Question 2- Geometrical shapes of the complexes formed by the reaction of
-
octahedral, tetrahedral and square planar
-
tetrahedral, square planar and octahedral
-
square planar, tetrahedral and octahedral
-
octahedral, square planar and octahedral
Show Answer
Answer: (2)
Hint: Reaction of
Reaction of
Reaction of
PRACTICE QUESTIONS
Question 1- Amongst the following, the lowest degree of paramagnetism per mole of the compound at 298
(a)
(b)
(c)
(d)
Show Answer
Answer:- bQuestion 2- Amongst
(a)
(b)
(c)
(d)
Show Answer
Answer:- cQuestion 3- Among the following ions, which one has the highest paramagnetism?
(a)
(b)
(c)
(d)
Show Answer
Answer:- bQuestion 4- Which of the following is formed when excess of
(a)
(b)
(c)
(d)
Show Answer
Answer:- dQuestion 5- The complex ion which has no d-electrons in the central metal atom is
(a)
(b)
(c)
(d)
Show Answer
Answer:- aQuestion 6- The compound having tetrahedral geometry is
(a)
(b)
(c)
(d)
Show Answer
Answer:- dQuestion 7- Spin only magnetic moment of the compound
(a)
(b)
(c)
(d)
Show Answer
Answer:- bQuestion 8- Among the following metal carbonyls, the
(a)
(b)
(c)
(d)
Show Answer
Answer:- bQuestion 9- Both
(a)
(b)
(c)
(d)
Show Answer
Answer:- bQuestion 10- Among the following, the coloured compound is
(a)
(b)
(c)
(d)
Show Answer
Answer:- c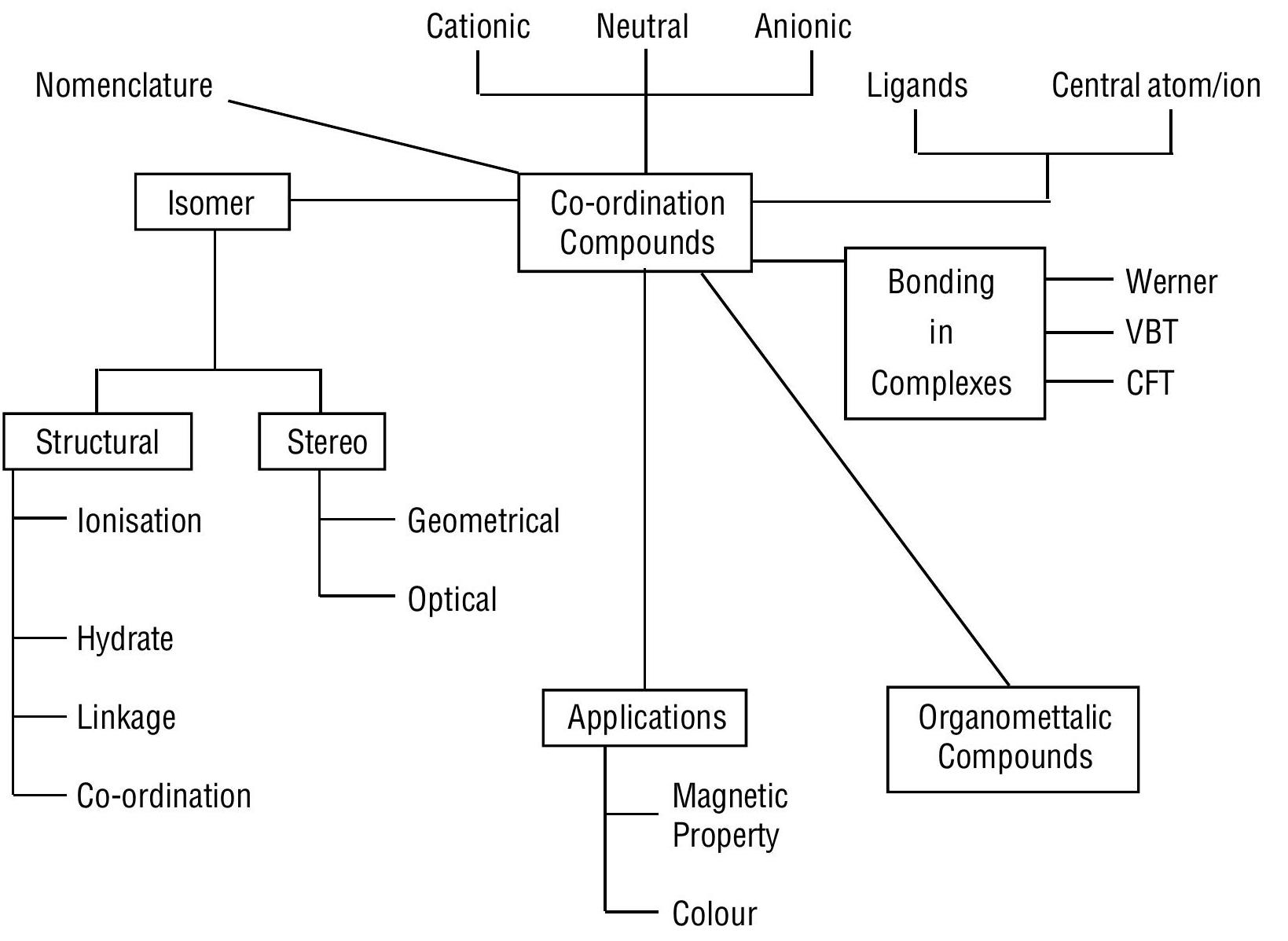