Unit 11 Some P Block Elements
GENERAL PRINCIPLES OF INORGANIC CHEMISTRY
Trends in the Periodic Table
Shells upto (
1. General electronic configuration is ( varies from 2 to 7 )
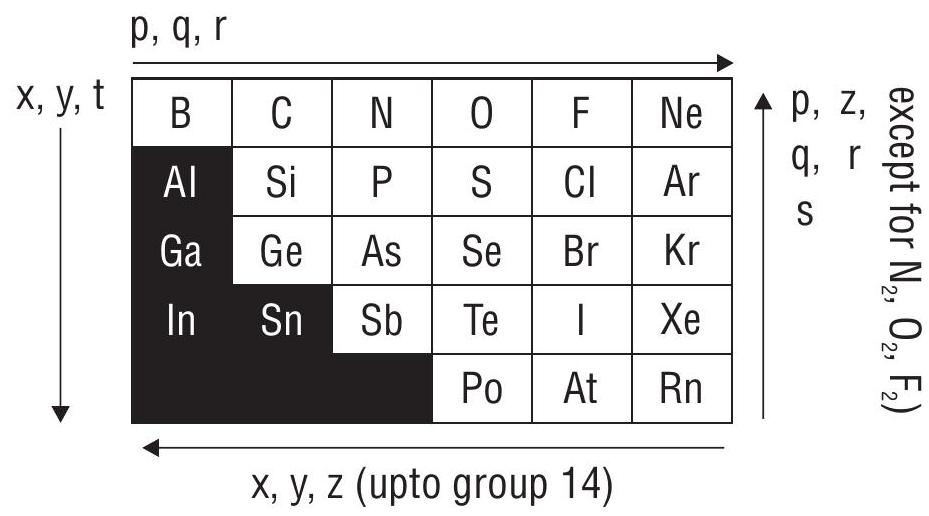
Where …
x: covalent/van der Waals’ radius
It: stability of lower oxidation state within a group.
Trends in Properties of -Block Elements in the direction of arrow
The highest oxidation state
Oxidation states
On descending the group, a lower oxidation state which is two units less than the highest oxidation state becomes more stable in group 13 to 16 . This trend is due to inert pair effect. For example, the highest oxidation state for the elements of group 13 is +3 . However, in addition to + 3 oxidation state, these elements also show +1 oxidation state which becomes more stable than +3 . Similarly, for group 14 , the group oxidation state is +4 , but +2 oxidation state becomes more stable on going down the group. For example, the last element, lead +2 oxidation state is more stable than +4 oxidation state. Similarly, thallium, +1 Oxidation State is more stable than +3 .
This trend of occurrence of oxidation state two units less than the group oxidation state is called inert pair effect and becomes more and more prominent as we move down the group.
The common oxidation states displayed by the p-block elements are given in Table 1.
Table 1. Common oxidation states of p-block elements
Group | 13 | 14 | 15 | 16 | 17 | 18 |
---|---|---|---|---|---|---|
General electronic configuration |
||||||
Group oxidation state | +3 | +4 | +5 | +6 | +7 | +8 |
Various oxidation states |
+3 +3 |
C +4 Ge, Sn, |
+5 to -3 |
0 |
-1 |
Metallic and non-metallic character
The p-block contains metallic and non-metallic elements. It is very interesting to note that the non-metals and metalloids exist only in the p-block of the periodic table. The non-metallic character increases along a period but decreases down a group. In fact the heaviest element in each p-block group is the most metallic in nature. Therefore, the elements with most metallic character are located mostly in the lower left portion while those with most non-metallic character are present at the top right portion of the periodic table. In between these, there are some elements which show characteristics of both metals and non-metals and are called metalloids. The common metalloids in p-block elements are B, Al, Si, Ge, As, Sb, Te, Po, At. This change from non-metallic to metallic brings significant diversity in the chemistry of these elements.
In general, non-metals have higher ionization enthalpies and higher electronegativities than metals. Therefore, in contrast to metals which readily form cations, non-metals readily form anions. The compounds formed by combination of highly reactive non-metals, with highly reactive metals are generally ionic in nature because of large differences in their electronegativities. On the other hand, compounds formed between non-metals themselves are largely covalent in character because of small differences in their electronegativities. It can be understood in terms of their oxides. The oxides of non-metals are acidic or neutral whereas oxides of metals are basic in nature. The oxides of metalloids are amphoteric. Further more, the more electropositive the metal, the more basic is it and the more electronegative the non-metal, the more acidic is its oxide. Therefore, in p-block elements, acidic character of the oxides increases or basic character decreases along a period. Similarly, the basic character of the oxides increases or acidic character decreases down the group.
Differences in behavior of first element of each group
The first member of each group of p-block differs in many respects from its succeeding members (called congeners) of their respective groups. For example, boron shows anomalous behaviour as compared to rest of the members of the 13 group elements. The main reasons for the different behaviour of the first member as compared to other members is because of
(i) small size of the atom and its ion
(ii) high electronegativity and
(iii) absence of d-orbitals in their valence shell
These factors have significant effect on the chemistry of first element as compared to other elements (specially second). For example
(a) Covalence upto four
First member of each group belongs to second period elements and have only four valence orbitals i.e., one
(i) Boron forms only
(ii) Carbon can form only tetrahalides (
(iii) Nitrogen forms only
(iv) Fluorine does not form
(b) Reactivity
Due to availability of d-orbitals of elements of third period, they are more reactive than elements of second period which do not have d-orbitals. For example, tetrahalides of carbon are not hydrolysed by water whereas tetrahalides of other elements of group 14 are readily hydrolysed.
The hydrolysis involves the nucleophilic attack of water molecule.
(c) Tendency to form multiple bonds
Because of the combined effect of smaller size and nonavailability of
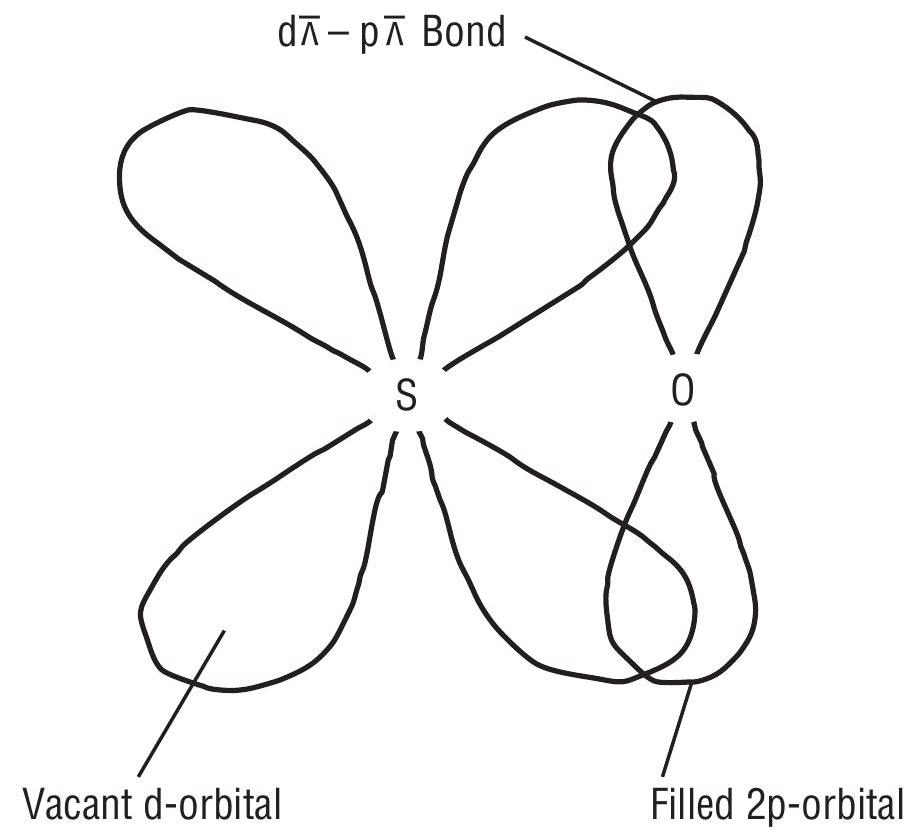
Because the
-bonding).
The first member of 13 group (boron) shows diagonal relationship with silicon (of group 14).
Group 13 and Group 14
Group 13
(elements) B, AI, Ga, In and TI,
The elements belonging to groups 13 to 18 belong to
B and AI have noble gas core, Ga and In have noble gas plus
General Trends in Properties
1. General electronic configuration
2.Atomic radius
‘Ga’ has smaller atomic size due to the poor shielding effect of the inner 3d electrons.
3.Ionization enthalpy (I.E.)
This is due to the poor shielding effect of the inner electrons.
The order of ionization enthalpies as expected is
4.Electro negativity
This is because of the discrepencies of their atomic sizes.
Baron is non metallic in nature. It is extremely hard and black coloured solid.
Density of the elements increases down the group from B to TI.
5. Melting point
6. Oxidation state
The general oxidation state of the
The relative stability of +1 oxidation state progressively increases for heavier elements
These elements in their trivalent state for electron deficient compounds which act as lewis acid for example
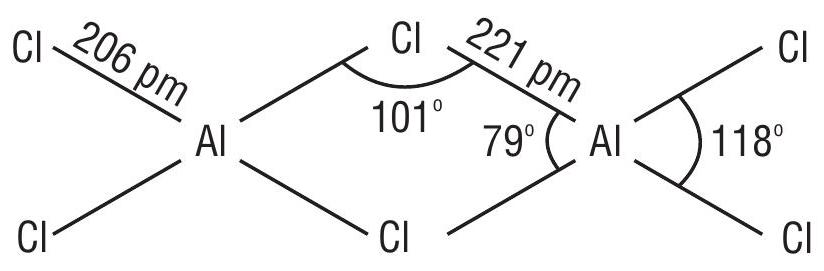
In trivalent state most of the compounds being covalent are hydrolysed in water.
Reactivity towards air
The nature of these oxides vary down the group.
Reactivity towards acids and alkalies
B does not react with acids and alkalies. Al dissolves in mineral acids and aqueous alkalies and thus shows amphoteric character
Reactivity towards Halogens
These elements react with halogens to form trihalides (except
Important trends and anomalous properties of boron
The trichlorides, bromides and lodides of all these elements being covalent in nature are hydrolysed in water.
The monomeric trihalides, being electron deficient are strong lewis acids.
Due to absence of
Boron
Minerals of boron
1. Borax
2. Boric acid
3. Colemanite
4. Kernite
Preparation of Boron
1. By thermal decomposition of boron hydrides
Boron can be prepared by the thermal decomposition of its hydrides such as diborane
2. Conversion of borax into boron trioxide
Borax is treated with hot and concentrated hydrochloric acid to convert it first into boric acid.
1. General properties of Boron
i)
ii)
iii)
2. Hydrides of Boron
Diborane
Preparation of
i)
ii)
Properties of diborane
i) It burns in oxygen and air to form
This is why diborane is used as a rocket fuel.
ii) It hydrolyses in water to form boric acid.
iii) Diborane reacts with ammonia at
Borazine has the following structure which is similar to that of benzene. Hence, borazine is also referred to as inorganic benzene. It is isoelectronic to benzene.
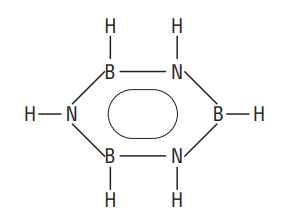
Structure of diborane
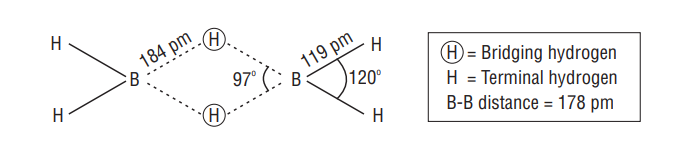
Boron Halides
This is due to
Although both
Boron also forms a series of hydridoborates the most important one is the tetrahedral [
Both
Boric Acid
Preparation
1. From borax
2. From colemanite
- Boric acid
contains triangular ions in which boron is hybridized. In solid state, molecules are -bonded to form a two-dimensional sheet. It is a weak monobasic acid. It does not act as a protonic acid but acts as a Lewis acid by accepting a pair of electrons from ion of water thereby releasing a proton.

- Reaction with ethyl alcohol. Orthoboric acid reacts with ethyl alcohol in presence of conc.
to form triethylborate.
The vapours of triethylborate when ignited burn with a green-edged flame. This forms the basis for detecting borates and boric acid in qualitative analysis.
Heating

Borax
White crystalline solid
Cyclic structure represented as
Aqueous solution is slightly alkaline due to hydrolysis
On heating borax, itloses water of crystallization and swells into white opaque mass.

Borax bead test
On heating, borax swells up forming a glassy mass of mixture of
Group-14-Carbon Family
Some physical constants of group 14 elements.
Atomic number | 6 | 14 | 32 | 50 | 82 |
Atomic mass | 12.01 | 28.09 | 72.60 | 118.71 | 207.2 |
Atomic radius (pm) | 77 | 118 | 122 | 140 | 146 | |
---|---|---|---|---|---|---|
Ionic radius (pm) | - | 40 | 53 | 69 | 78 | |
- | - | 73 | 118 | 119 | ||
Ionisation enthalpy | 1086 | 756 | 761 | 708 | 715 | |
(kJ mol |
||||||
2352 | 1577 | 1537 | 1411 | 1450 | ||
4620 | 3228 | 3300 | 2942 | 3081 | ||
6220 | 4354 | 4409 | 3929 | 4082 | ||
2.5 | 1.8 | 1.8 | 1.8 | 1.9 | ||
Electronegativity | 3.51 | 2.34 | 5.32 | 7.26 | 11.34 | |
Density |
4373 | 1693 | 1218 | 505 | 600 | |
m.p. (K) | - | 3350 | 3123 | 2896 | 2024 | |
b.p. (K) | 50 | 50 | ||||
Electrical resistivity | 50 | |||||
(ohm cm at 293 K) |
1. Atomic Radius
There is a small increase in radius due to presence of completely filled
2. Ionization energy
The influence of inner core electrons is present in group 14 also.
Small decrease in
3. Electro negativity
Due to small size the elements, this group elements are slightly more electronegative than group 13 elements. The electronegativity values for elements from
4. Melting Point
Oxidation state
The common oxidation states exhibited by these elements are +4 and +2 .
The sum of the first four ionization enthalpies is very high. In heavier members the tendency to show +2 oxidation state increases in the sequence
Reactivity towards oxygen
All members form oxides of two types monoxide and dioxide (
Among monoxides
Reactivity towards water
Lead is not affected by water.
Reactivity towards halogen
They form halides of formula
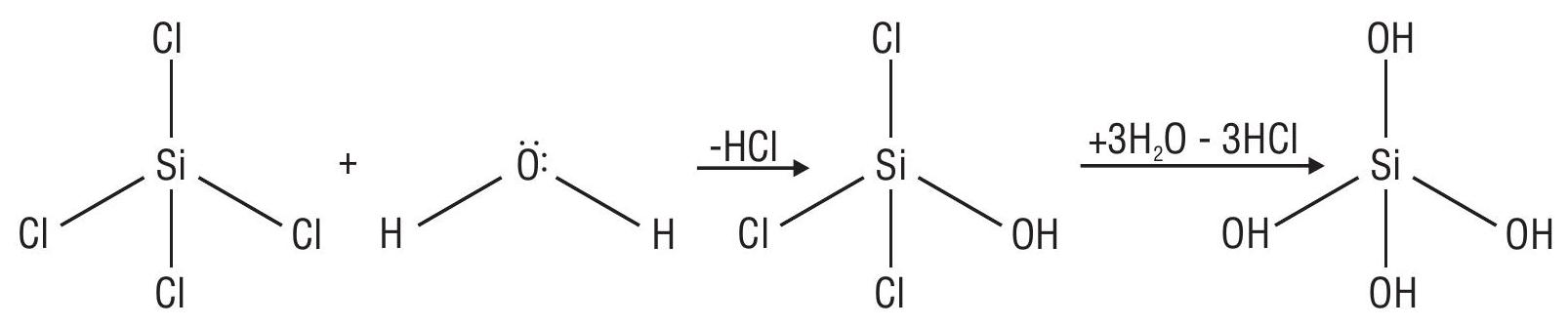
Allotropes of Carbon
1. Diamond
Three dimensional network of carbon atoms joined through strong covalent bonds.
Each
Hardest substance known
Very high melting point (3843 K)
Bad conductor of electricity since all valence electrons are involved in bond formation.
Transparent and has high refractive index (2.45)
2. Graphite
Each carbon atom is
Planar hexagonal rings are formed.
They are held together by weak vanderwaal’s forces.
Sheets can slide over each other
Soft and has lubricating properties.
3. Silica (Silicondioxide)
(a) Structure
Covalentcompound
Si is tetrahedrally surrounded by 4 oxygen atoms
Each oxygen is shared by two silicon atoms
Covalent bonds between
(b) Physical properties
Insoluble in water
Does not react with acids
High melting point
(c) Chemical properties
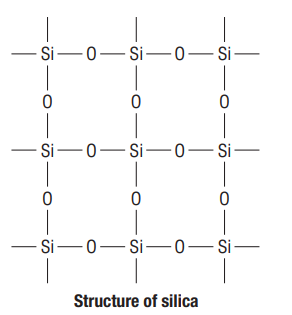
4. Silicates
Contain
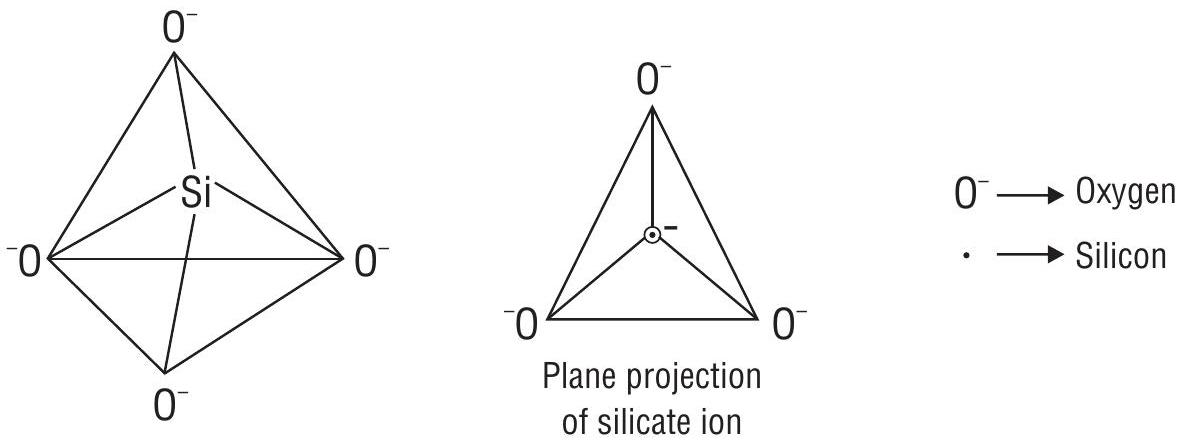
Classification of silicates
Based on the way
(a) Orthosilicates
Contain single discrete unit of
(b) Pyrosilicates
Contain two units of
Pyrosilicate ion is
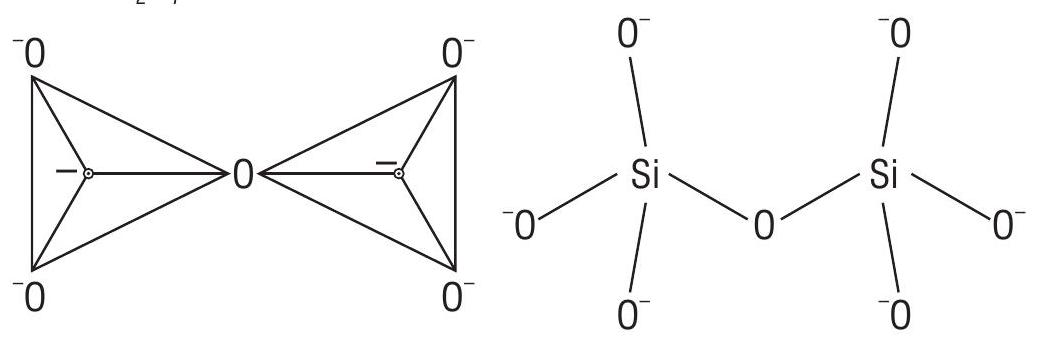
(c) Cyclic structure - Cyclic or ring silicates have general formula
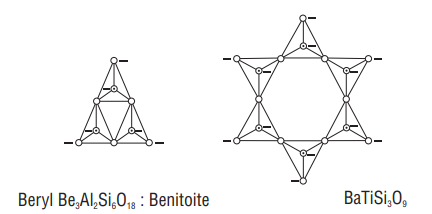
(d) Chain silicates
If two oxygen atoms per tetrahedron are shared such that a linear single strand chain of the general formula
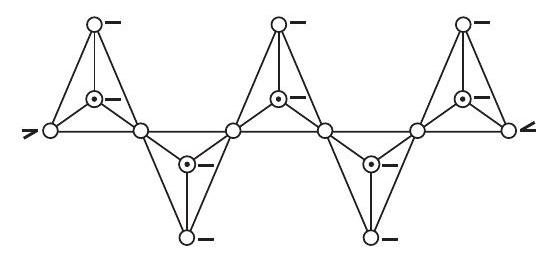
(e) Sheet silicates
Three oxygen atoms of a tetrahedral
(f) Three dimensional sheet silicates
These silicates involve all four oxygen atoms in sharing with adjacent
5. Silicones
Silicones are synthetic organosilicone polymers containing repeated
Linear Chain silicones are obtained by hydrolysis of
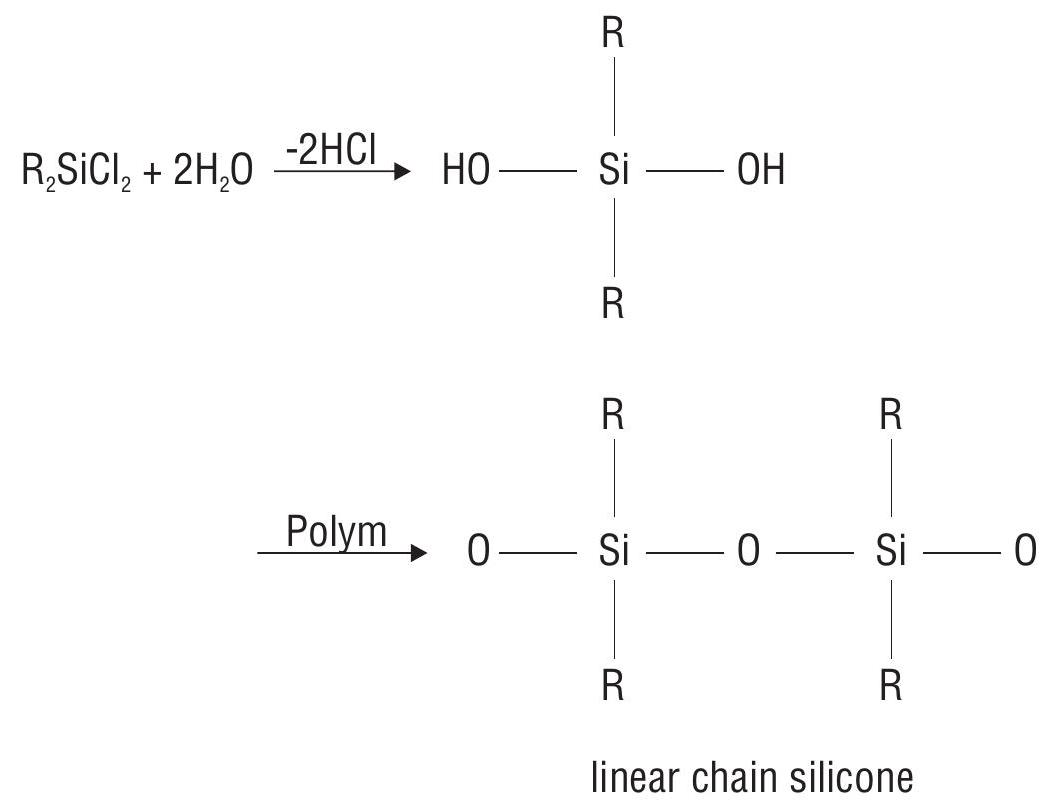
If
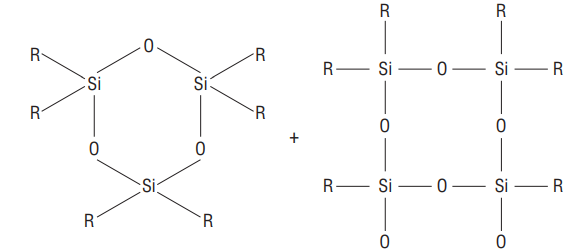
Some
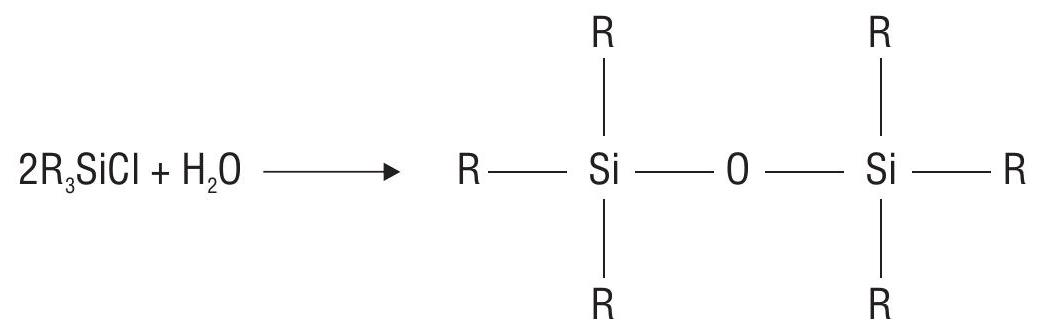
ii) Cross-linked silicones
Hydrolysis of
6. Zeolites
The three-dimensional silicates in which some of the
Zeolites are widely used as catalysts in petrochemical industries for cracking of hydrocarbons and
isomerization. Another zeolite called ZSM-5 is used to convert alcohols directly into gasoline. Hydrated zeolites called permutitare used as ion exchangers for softening of hard water.
Solved Examples
1. Which one of the following is the correct statement?
-
Boric acid is a protonic acid.
-
Beryllium exhibits coordination number of six.
-
Chlorides of both beryllium and aluminium have bridged chloride structure in solid phase.
-
is known as inorganic benzene.
Show Answer
Answer: 3
Rest 3 statements are incorrect. Basic acid is a non protic acid.
Beryllium exhibits coordination number of 4.
Borazine is
2. A metal,
-
is more volatile than . -
is more soluble in anhydrous ethanol than . -
is more ionic than . -
is more easily hydrolysed than .
Show Answer
Answer: 3
3. In silicon dioxide
-
Each silicon atom is surrounded by four oxygen atoms and each oxygen atom is bonded to two silicon atoms.
-
Each silicon atom is surrounded by two oxygen atoms and each oxygen atom is bonded to two silicon atoms.
-
Silicon atom is bonded to two oxygen atoms.
-
There are double bonds between silicon and oxygen atoms.
Show Answer
Answer: 1Practice Questions
1.
(a) monobasic acid and weak lewis acid
(b) monobasic and weak bronsted acid
(c) monobasic and strong Lewis acid
(d) tribasic and weak Bronsted acid
Show Answer
Answer: (a)2. (Me)
(a)
(b)
(c)
(d)
Show Answer
Answer: (c)3. Name of the structure of silicates in which three oxygen atoms of
(a) pyrosilicate
(b) sheet silicate
(c) linear chain silicate
(d) three dimensional silicate
Show Answer
Answer: (b)4.
(a) Addition of cis 1,2 diol
(b) Addition of borax
(c) Addition of trans 1,2 diol
(d) Addition of
Show Answer
Answer: (b)5. The product/s formed when diborane is hydrolysed is/are
(a)
(b)
(c)
(d)
Show Answer
Answer: (d)6. Reaction of diborane with ammonia gives initially
(a)
(b) Borazine
(c)
(d)
Show Answer
Answer: (b)7. The structure of diborane
(a) four 2c-2e bonds and two
(b) two 2c-2e bonds and four
(c) two 2c-2e bonds and two
(d) four 2c-2e bonds and four 3c-2e bonds
Show Answer
Answer: (a)8. In borax, the number of
(a) five and four
(b) four and five
(c) three and four
(d) five and five
Show Answer
Answer: (b)9. Which one of the following has highest Lewis acid strength?
(a)
(b)
(c)
(d)
Show Answer
Answer: (a)10. Which one of the following is the correct statement?
(a)
(b) Boric acid is a protonic acid.
(c) Beryllium exhibits coordination number of six.
(d) Chlorides of both beryllium and aluminium have bridged chlorine structure in solid phase.
Show Answer
Answer: (d)